Research
Coherent Ising Machine
A coherent Ising machine (CIM) is a network of laser oscillators designed to find ground states of the Ising model based on the gminimum-loss principleh
proposed by Y. Yamamoto and coworkers [1].
In a CIM, the problem, or spin-spin coupling terms of the Ising model are mapped onto the amplitude and phase of the optical couplings
between the gartificial spinsh, represented by the phases of laser oscillators [1] or degenerate parametric oscillators (DOPO) [2,3].
By pumping the coupled oscillators slowly, the oscillator network tends to start oscillating at a phase configuration that minimize the total loss,
that corresponds to the ground state of the Ising model represented by the oscillators.
In 2013, we started constructing time-multiplexed DOPOs using a long-distance (1 km) fiber cavity, where >2500 DOPOs were successfully obtained
using dual-pump four-wave mixing in a nonlinear fiber as a phase sensitive amplifier (PSA) placed in a cavity [4].
This experiment was followed by the construction of DOPOs using a PPLN waveguide-based PSA [5], and further increase of the DOPO numbers to >10,000 [6,7].
These experiments paved a way towards a realization of a scalable CIM.
Currently, the number of DOPOs generated by a single cavity increased to 50,000 with a phase stabilized cavity and >1 million with a free-run cavity [8].
In addition, we implemented one-dimensional Ising model using optically-coupled DOPOs,
and confirmed that the networked DOPOs well simulated the behavior of low-temperature spins [7,9,10].
In 2016, we realized a 2048-node CIM based on the measurement-feedback scheme [11], where the spin-spin coupling are achieved
by feedbacking the signal obtained from DOPO quadrature measurement to each DOPOs circulating in the cavity [12].
The CIM with the measurement-feedback scheme successfully found solutions to 2000-node maximum cut problems with less than a ten-thousandth of a second [11].
This research is financially supported by the ImPACT Program, where I serve as the Principal Investigator of the project named gCoherent Ising Machine based on large-scale time-multiplexed degenerate optical parametric oscillatorsh.
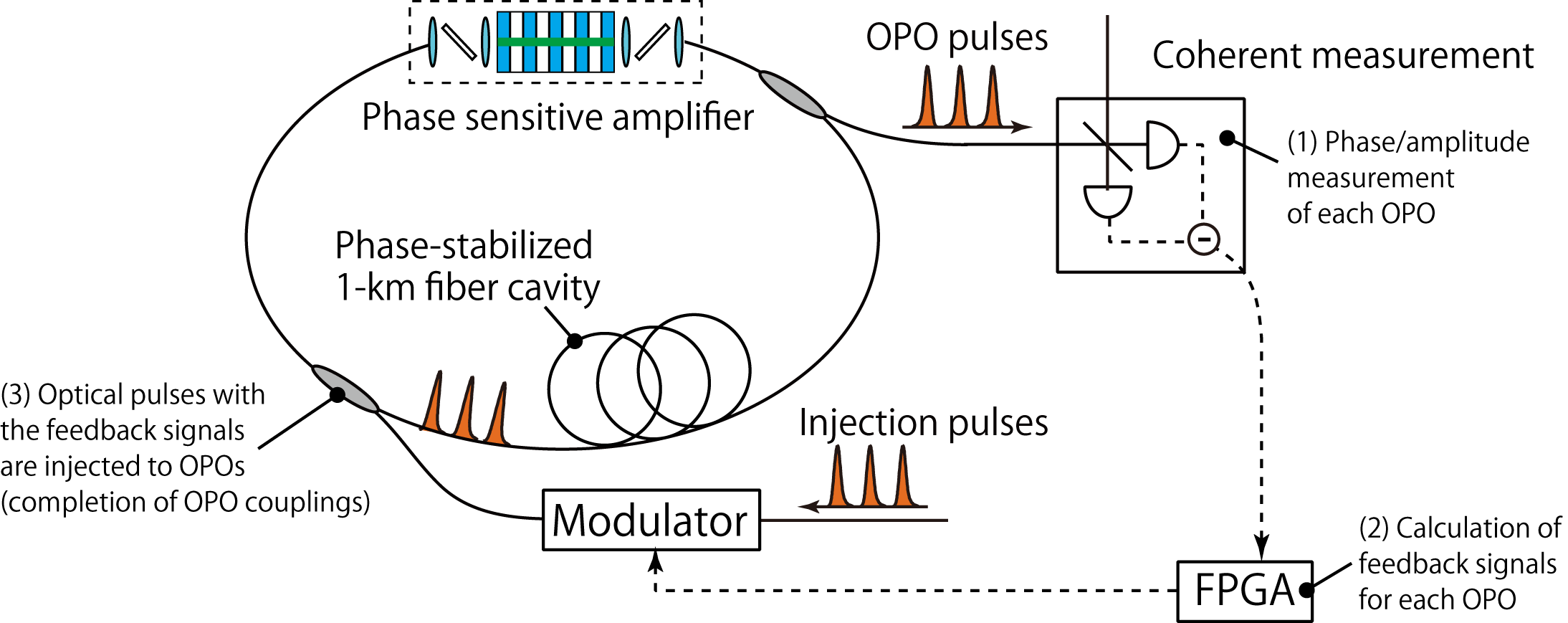
Fig: Coherent Ising machine with measurement-feedback.
- S. Utsunomiya, K. Takata, and Y. Yamamoto, gMapping of Ising models onto injection-locked laser systems,h Opt. Express 19, 18091 (2011).
- Z. Wang, A. Marandi, K. Wen, R. L. Byer, and Y. Yamamoto, gCoherent Ising machine based on degenerate optical parametric oscillators,h Phys. Rev. A 88, 063853 (2013).
- A. Marandi, Z. Wang, K. Takata, R. L. Byer, and Y. Yamamoto, gNetwork of time-multiplexed optical parametric oscillators as a coherent Ising machine,h Nature Photonics 8, 937 (2014).
- H. Takesue, T. Inagaki, K. Inoue, and Y. Yamamoto, "Coherence property of >2500-pulse multiplexed degenerate OPO,"
Japan Society of Applied Physics Spring Meeting 2014, 17p-PA1-1 (March 17, 2014).
- H. Takesue, T. Inagaki, T. umeki, O. Tadanaga, and H. Takenouchi,"Large-scale time-division multiplexed OPOs using PPLN waveguide,"
Japan Society of Applied Physics Spring Meeting 2015, 12a-P6-1 (March 12, 2015).
- H. Takesue, T. Inagaki, K. Inoue, and Y. Yamamoto, "Time-division-multiplexed degenerate optical parametric oscillator for a coherent Ising machine," IEEE Summer Topicals 2015, TuF4.2, July 14, 2015, Nassau, Bahamas (invited).
- T. Inagaki, K. Inaba, R. Hamerly, K. Inoue, Y. Yamamoto, and H. Takesue, "Large-scale Ising spin network based on degenerate optical parametric oscillators," Nature Photonics 10, 415-419 (2016).
- H. Takesue and T. Inagaki, "10 GHz clock time-multiplexed degenerate optical parametric oscillators for a photonic Ising spin network," Opt. Lett. 41, 4273-4276 (2016).
- T. Inagaki, K. Inoue, Y. Yamamoto, and H. Takesue, "Simulating one-dimensional Ising spins with optically-coupled timedivision-multiplexed optical parametric oscillators," Nonlinear Optics (NLO) 2015, NTu1B.6, July 28, 2015, Kauai, Hawaii.
- T. Inagaki, K. Inaba, H. Takesue,
"Simulating 1-dimensional Ising model with an OPO network," Japan Society of Applied Physics Fall Meeting 2015, 15a-4D-3iSeptember 15, 2015j.
- T. Inagaki, Y. Haribara, K. Igarashi, T. Sonobe, S. Tamate, T. Honjo, A. Marandi, P. L. McMahon, T. Umeki, K. Enbutsu, O. Tadanaga, H. Takenouchi,
K. Aihara, K. Kawarabayashi, K. Inoue, S. Utsunomiya, and H. Takesue, "A coherent Ising machine for 2000-node optimization problems," Science (2016)
- S. Utsunomiya, Y. Yamamoto, H. Takesue, PCT/JP2015/059057
Quantum Communication and integrated quantum photonics
- Telecom-band entangled photons (Fig. 1,2).
- Quantum frequency conversion (Fig. 3).
- Differential phase shift quantum key distribution (Fig. 4, 5).
- Silicon photonics quantum information (Fig. 6).
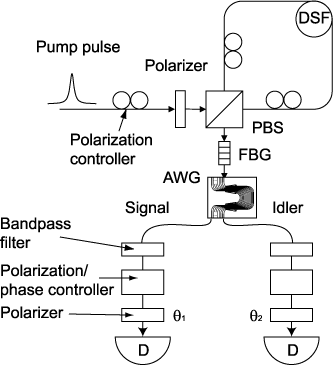
Fig.1: Configuration for generating polarization entanglement using spontaneous four-wave mixing (SFWM) in dispersion shifted fiber (DSF).
H. Takesue and K. Inoue, Phys. Rev. A 70, 031802(R) (2004).
Fig.2: Configuration for generating time-bin entanglement using SFWM in cooled DSF. The DSF was cooled to suppress noise photons caused by
spontaneous Raman scattering. H. Takesue and K. Inoue, Phys. Rev. A 72, 041804(R) (2005): H. Takesue and K. Inoue, Opt. Express, 13, 7832 (2005).
Fig.3: Frequency up-conversion single photon counter. C. Langrock et al., Opt. Lett., 30, 1725 (2005).
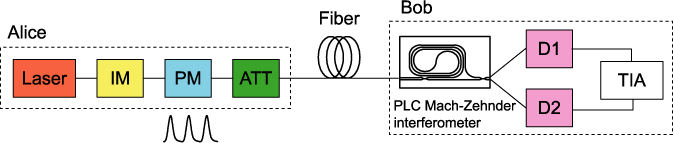
Fig.4: Configuration of differential phase shift quantum key distribution (DPS-QKD).
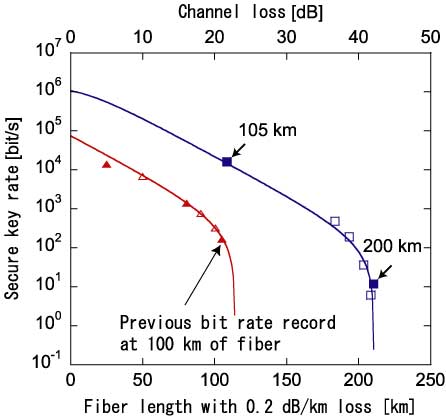
Fig.5: Experimental result of DPS-QKD: secure key generation rate as function of transmission fiber length.
Secure keys with a bit rate of 12 bit/s was successfully generated over 200 km of fiber.
H. Takesue et al., Nature Photonics 1, 343 (2007).
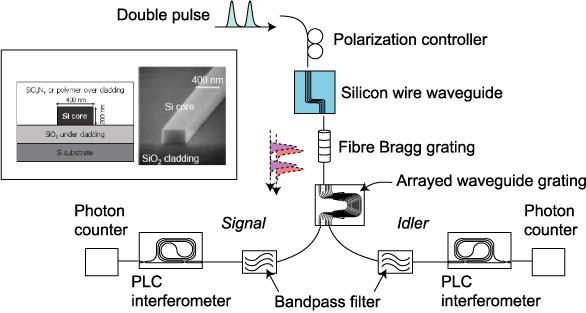
Fig.6: The setup of the first experimental entanglement generation using a silicon waveguide.
Inset: the silicon wire waveguide used for the experiment fabricated by NTT Microsystem Integration Laboratories. H. Takesue et al., Appl. Phys. Lett. 91, 201108 (2007).
Wavelength-Division Multiplexing (WDM) Access Networks
- WDM access ring using light source centralization.
- Data rewriting of wavelength channels
using saturated semiconductor optical amplifier.
Lightwave Frequency Synthesis and Its Applications
- Broadband lightwave frequency sweeper using synchronous filtering.
- Stabilization of lightwave frequency sweeper using Fabry-Perot cavity
composed of Faraday rotator mirrors.
- Chromatic dispersion measurement using lightwave frequency sweeper.
Home