Electron spin resonance spectroscopy using a superconducting flux qubit featuring high sensitivity and spatial resolution
- Highly sensitive detection of electron spins in a micrometer scale area -
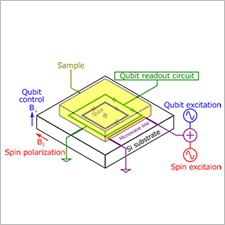
NTT Basic Research Laboratories (NTT-BRL) have realized electron spin*1 resonance*2 (ESR) spectroscopy using a superconducting flux qubit*3 for a small number of electron spins in a tiny sample volume. ESR spectroscopy is the typical method to characterize electron spins in materials and commonly used to analyse molecular structures, for example. However, the required number of spins (~1013 spins) and sample volume (~[1 cm]3) needed for analysis in a conventional ESR spectrometer*4 is large. Thus, there is limitation for the sample to be analysed.
A superconducting flux qubit works as a sensitive magnetometer. Here, we show that the magnetometer can detect electron spins (which themselves can be considered to be small magnets). In our method, we can detect 400 electron spins in 0.05 pL (~[4 μm]3) sensing volume. The development of our new ESR spectrometer for a small number of electron spins in small volume will contribute to wide range of fields from basic science to material characterization including biomaterials and medical diagnosis. This research was reported in Communications Physics on March 29, 2019.
This work was supported by CREST (JPMJCR1774), JST.
=> Press Release
=> Superconducting Quantum Circuit Research Group
Background
Electron spin resonance (ESR) spectroscopy is the most commonly used method to analyse samples containing electron spins (unpaired electrons). However, a large number of spins (~1013 spins) and large volume of sample (several mL) has been required for such analysis, so limiting the area’s in which it can be applied.
In this investigation, we improve the sensitivity and spatial resolution (sensing volume) of an ESR spectrometer by using a superconducting flux qubit as a sensitive magnetometer. This enables us to expand the application area of ESR spectroscopy.
Achievements
Researchers of NTT-BRL have performed ESR spectroscopy with a superconducting flux qubit, which consists of an aluminium superconducting circuit (Fig. 1). The achieved sensitivity is 400 spin using sensing volume of 0.05 pL with one second signal averaging time. This enables us to realize an ESR spectrometer for a small number of electron spins with microscale spatial resolution.
The experiment is performed below 20 mK (close to absolute zero temperature). A spin ensemble is directly attached to the flux qubit chip. An in-plane external magnetic field is applied to the sample. The electron energy level splits into two for up spins and down spins by this field (Fig. 2). In this configuration, electron spins which are parallel to the in-plane field are energetically stable, and most of the spins align to the direction of the in-plane field. The electron spins generate a magnetic field if they are aligned because electron spins can be treated as small magnets. We measure this magnetic field by the superconducting flux qubit. The flux qubit is very sensitive to the magnetic flux penetrating through the loop structure (Fig. 1, blue false coloured area). We then apply a microwave signal to the electron spins whose energy is same as the energy splitting of the electron spins. Due to the resonance phenomenon with the microwave signal, some of the spins flip and direct anti-parallel direction to the in-plane field. Thus, the magnetic field from up and down spins cancels out and the total magnetic field generated by the electron spins becomes weaker. By using this principle, we detect the ESR signal by measuring the magnetic field generated by the electron spins. In our ESR experiment, we sweep the frequency (energy) of the microwave to find out the resonance frequency, and we can obtain the energy splitting between the up and down spins. We can extract material parameters from the energy splitting.
We use nitrogen vacancy (NV) centres in diamond*5 as our sample for this experiment (Fig. 3). We sweep the frequency (energy) of the microwave signal for the spin excitation under the in-plane filed of 5.8 mT. We measure the magnetic field by the superconducting flux qubit and observe two large peaks in the ESR spectrum ( Fig. 4). These two peaks reflect the electron state of the NV centre. We can extract the material parameters from the peak positions, which show good agreement with the literature*6.
Technical features
- Improvement of the sensitivity with a superconducting flux qubit
A superconducting flux qubit shows 1000 times better responsivity than a conventional highly sensitive magnetometer, superconducting quantum interference device (SQUID). The sensitivity of the ESR spectrometer is quantified by comparing the noise from an experimental setup and a measured signal. We evaluate the noise from our ESR spectrometer and conclude that the sensitivity corresponds to 400 spins for a signal integration time of one second. - Improvement of the spatial resolution with a superconducting flux qubit
We can control the loop size of the superconducting flux qubit by a design. The spatial resolution of our ESR spectrometer is determined by the loop size of the flux qubit. A superconducting flux qubit with 24 × 2 μm loop structure is used for our experiment (Fig. 1). This loop size corresponds to the sensing volume of 0.05 pL. Further improvement of the spatial resolution is possible by shrinking down this loop size. - Two parameter sweep with a non-resonant type ESR spectrometer
In conventional ESR spectrometers, a sample is enclosed in the cavity and a microwave signal which resonates with the cavity is applied. The ESR signal is detected by sweeping the magnetic field. From this intrinsic feature of the spectrometer, it is difficult to perform ESR spectroscopy far from the cavity resonance frequency. On the other hand, we use a superconducting flux qubit as a magnetometer to perform ESR spectroscopy. We have no limit on the applicable microwave frequency and so can perform ESR spectroscopy by sweeping two parameters: the microwave frequency and the magnetic field (Fig. 4). By sweeping these two parameters, we can obtain an ESR spectrum with much wider range, which in turn enables us to refine material parameters.
Future plans
We will further improve the sensitivity of the ESR spectrometer by optimizing our measurement system and the flux qubit. We are also developing a superconducting flux qubit array for spatial imaging of the ESR signal.
Publication information
Hiraku Toida, Yuichiro Matsuzaki, Kosuke Kakuyanagi, Xiaobo Zhu, William J. Munro, Hiroshi Yamaguchi, and Shiro Saito
“Electron paramagnetic resonance spectroscopy using a single artificial atom”
Communications Physics 2, 33 (2019).
Glossary
*1 ... Electron spin
Materials are composed of atoms as basic building blocks. An atom is composed of nuclei at the centre and surrounding electrons. The electron rotates like the earth or moon. The rotation of the electron is called electron spin. The rotation axis of the electron spin is not fixed in general and can move due to the interaction with external fields including a magnetic field.
*2 ... Electron spin resonance (ESR)
Magnetic resonance imaging (MRI) is a commonly used technique in medical diagnosis. This method visualizes the properties of nuclei in the body. On the other hand, electron spin resonance is a method to characterize the electron spins in a sample.
*3 ... Superconducting flux qubit
A superconducting flux qubit is a superconducting loop containing multiple Josephson junctions. Under a magnetic flux bias, clockwise and counter clockwise current can be treated as eigenstates of the two-level system. We can use the superconducting flux qubit as a magnetometer to detect electron spins, because the magnetic field generated by electron spins can be treated as an external magnetic flux bias.
*4 ... Electron spin resonance (ESR) spectrometer
A conventional electron spin resonance spectrometer, which is used to analyse materials, consists of a microwave cavity (metal box), a measurement system of microwave transmittance and strong electromagnets. The sample is inserted to the cavity. The microwave transmittance is measured as a function of a magnetic field with a microwave signal which is resonant with the cavity. The energy splitting of the electron spin increases as increasing the magnetic field strength. If the microwave energy resonates with the energy splitting of the electron spin, peaks should appear in the microwave transmittance. The peaks contain the information of electron spins, thus material properties are extracted by analysing the peak position (qualitative figure) and the peak height (quantitative figure).
*5 ... Nitrogen-vacancy (NV) centre
The nitrogen-vacancy (NV) centre is considered to be the origin of the colour of pink diamonds. The NV centre is an impurity defect that consists of a nitrogen (N) atom and a vacancy (V) substituting an adjacent carbon atom. We perform ESR spectroscopy on negatively charged NV centres.
*6 ... Reference for the material parameters of nitrogen-vacancy (NV) centres
We refer to the following literature for the material parameters of NV centres.
J. H. N. Loubser & J. A.v. Wyk
Electron spin resonance in the study of diamond
Rep. Prog. Phys. 41, 1201–1248 (1978)
In this literature, a standard ESR spectrometer is used to derive the material parameters. We compare the g-factor (g) and zero-field splitting (D) as follows.
Our results: g = 1.996 ± 0.013, D = 2.88071 ± 0.00087 GHz
Reference values: g = 2.0023 ± 0.005, D = 2.878 ± 0.006 GHz
The agreement between our results and the reference is well within the range of error bars.