The world's first realization of an optomechanical device with extremely low optical energy loss
- Progress toward the creation of smaller and more efficient optical amplification devices -
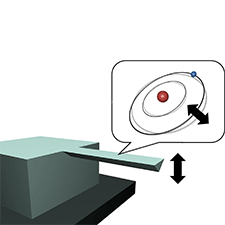
Researchers at NTT Basic Research Laboratories have sucessfully realized an optomechanical device ※3 with extremely low light energy loss by embedding rare-earth light-emmiting centers※2 inside a microscopic mechanical oscillator※1.
In conventional optomechanical devices, light couples to sound where the relaxation time ※4 of that light is usually much shorter than the corresponding relaxation time of the mechanical oscillator. In this regime it is possible to control and manipulate the mechanical oscillations using optical resonances. For technological applications, however, it is often desirable to achieve the opposite situation, where one uses the mechanical oscillations to control the light. Despite numerous experimental efforts, it has remained technologically challenging to acheive.
In our work, we have designed and experimentally realized a novel optomechanical device by coupling rare-earth emmiting centers to a mechanical resonator. Our device works in an unconventional limit: the relaxation time of the rare-earth emmiting centers is extremely long in comparison to the relaxation time of the mechanical motion. This is known as the reverse dissipation regime. Previous theoretical works have shown that in this regime it is possible to control light using the mechanical oscillation and to even to amplify that light using the optomechanical elements. We envision that our experimental results will lead to the development of compact, highly-efficient, and energy-saving optical devices such as on-chip optical amplifiers using small optomechanical elements with large non-linearities.
The results were published in "Physical Review Letters" on January 29, 2021.
=> Press Release
=> Nanomechanics Research Group
Background and summary of the results.
Micro-mechanical oscillators in semiconductor chips are used in diverse technological applications including high-sensitivity sensors and high-frequency filters to mention but a few. Among micro-mechanical oscillators, MEMS (Micro - Electro - Mechanical - Systems) are of utmost importance for technological applications because they can be controlled using electrical signals. Recently, there has been an increasing interest (both experimental and theoretical) of systems where light couples to sound, a research field known as optmechanics. Most optomechanical devices consist of a mechanical oscillator coupled to an optical resonator in which light is spatially confined by mirrors / holes, or an optical resonance in which light is selectively absorbed. The behavior of such optomechanical devices is determined by the ratio between the relaxation time of the light compared to the mechanical oscillation. Specifically, a physical system with a short relaxation time can be used to control another physical system with long relaxation time. In conventional optomechanical devices, the relaxation time of the light is extremely short compared to that of the mechanical oscillation. Thus, one can exploit this feature to control mechanical oscillations using light. On the other hand, the technological challenge has been to control light using mechanical oscillations (sound). In our work we have overcome this difficulty for the first time. We have fabricated a new optomechanical device in which the ratio between the relaxation time of the mechanical oscillation and light is reversed. We achieved this by embedding rare-earth emmiting centers with exceptionally long relaxation time into the mechanical oscillator. This will open a new avenue of research as one can use those mechanical oscillations to amplify and modulate optical resonances in the light-emitting centers. This has been extremely difficult to achieve in the past with other platforms.
Outline of the experiment
The mechanical oscillator used in the experiment (Fig. 1) was fabricated by milling YSO crystals※5 containing Erbium atoms (a rare-earth element). By placing the mechanical oscillator on top of a piezoelectric actuator, it was possible to electrically induce vertical oscillations and make the mechanical oscillator resonate at a natural frequency. This resonance allows one to induce a local strain inside the mechanical oscillator. By constructing an experimental system that can measure light absorption and emission depending on that strain, we successfully observed a strain-mediated interaction between the rare-earth emmiting centers and the mechanical oscillator (Fig. 2). Subsequent measurements of the energy loss confirmed that the relaxation time of the rare-earth emmiting centers is exceptionally long compared to that of the mechanical oscillator (Fig. 3).
Key technologies
- To efficiently couple light to a mechanical oscillator, light must be confined tightly in space for a long time. In conventional optomechanical devices, optical resonators※6 and quantum dots in semiconductors※7 were used as mechanisms to spatially confine that light. However, the optical relaxation time in those devices is short (on the order of few nanoseconds). This is extremely fast compared to the mechanical oscillators relaxation time. In our work, we have successfully fabricated a novel optomechanical device in which light remains confined in a tight space for time scales much longer than the relaxation time of the mechanical oscillator. This achievement was possible by embedding rare-earth emmiting centers whose relaxation time was several milliseconds into the mechanical oscillator. This not only improves the efficiency of coupling between the light field and mechanical oscillator, but also opens the possibility to amplify and manipulate light using those mechanical oscillations (this has been difficult to achieve with the conventional devices).
- The relaxation rate of these rare earth atoms embedded in solids is extremely long. Furthermore, crystals such as YSO containing rare earth elements are hard materials meaning it has been difficult to fabricate mechanical structures with them. We have overcome this difficulty by performing oblique milling using ion beams※8. For the first time in the literature we have successfully fabricated a mechanical oscillator coupled to rare-earth emmiting centers. By cooling the sample to liquid helium temperature (4K), we have quantitatively determined the interaction strength between the mechanical oscillator and the long-lived rare-earth emmiting centers.
Future development
Our work will open new avenues of research in this field. In the near future, we can use our optomechanical device that we have fabricated to demonstrate optical amplification and manipulate that light using mechanical oscillations. We will optimize the device structure so that the amplified light can be efficiently extracted. Further, while at present we have only demonstrated its operation at liquid helium temperatures, we aim our device to operate at both liquid nitrogen (77 K) and room (298 K) temperatures with the goal of using it within the framework of IOWN. We also aim to create energy-efficient optical devices that combine elastic waves and sound waves by increasing the interaction strength between the light and mechanical oscillator.
Paper publication information
"Rare-earth-mediated opto-mechanical system in the reversed displacement regime",
Ryuichi Ohta, Loïc Herpin, Victor M. Bastidas, Takehiko Tawara, Hiroshi Yamaguchi, and Hajime Okamoto,
Physical Review Letters 126, 047404 (2021)
Glossary
- *1 ... *1 Mechanical oscillators
- These provide an artificial structure in which mechanical oscillation is sustained by periodically applying elastic deformation. Vibration plates of musical instruments such as bells and iron harps are well known mechanical oscillators. In recent years, with the development of microfabrication technology, it has become possible to integrate mechanical oscillators (that are thinner and smaller than a human hair) into semiconductor chips. There are many proposals for the realization of practical MEMS oscillators. The most typical shape for these mechanical oscillators is the cantilever beam used in this study. This has a shape similar to a diving board in a pool.
- *2 ... Light-emitting centers
- In a solid-state material, there are electrons that remain isolated from their surroundings. These isolated electrons are called light-emitting centers because they absorb and emit light. Light-emitting centers can be used to design and fabricate lasers and photodetectors. In addition, when the coherence time of the emitters is long, they also can be used to build optical memories. Solid-state materials such as semiconductors, diamond, rare-earth elements, and a variety of other materials can host light-emitting centers.
- *3 ... Optomechanical devices
- These include an element having a structure in which the mechanical oscillation is affected by light or vice versa. An optical resonator may be used to enhance the interaction between light and the mechanical oscillator. With the use of optical resonators, light is confined in space thus enhancing the coupling to the mechanical motion. Instead of optical resonators, optical resonances of electronic levels in atoms may be embedded into mechanical oscillators.
- *4 ... Relaxation time
- The energy confined in both the mechanical oscillator and the light-emitting centers is gradually lost over time. The time required for that energy to be lost is called the energy relaxation time. In optomechanical devices, the characteristics of the device is determined by the relationship between the relaxation time of both the light and the mechanical oscillator. The factors that determine the relaxation time vary, but mechanical oscillators are often dependent on their structure while the light-emitting center is determined by the nature of the material that hosts it.
- *5 ... YSO crystal
- This solid-state crystal is a rare earth oxide. It can contain light-emitting centers of rare earth elements inside the crystal. Because YSO crystals are chemically stable, they are characterized by very little energy loss from the emittng centers. On the other hand, YSO crystals are very hard and difficult to fabricate with. Their application has typically been limited to optical devices that do not require fabrication of the crystal.
- *6 ... Optical resonators
- This is a structure that confines light spatially using reflection or refraction. It can utilize multiple reflections of light between mirrors or be a ring like structure with the light circulating around it. This is applied to the design and fabrication of lasers and optical filters.
- *7 ... Quantum dots
- This is a fine structure fabricated in a semiconductor that is widely used as a light-emitting center. The combination of semiconductor materials and the size of the microstructure can control the emission wavelength and emission efficiency. It is often used in LEDs and lasers.
- *8 ... A milling method using ion beams
- This is a processing method in which the material is physically removed by bombarding it with ions in vacuum. This method can be used to process various materials regardless of their composition. It is possible to create three-dimensional structures like a mechanical oscillator by firing the ions at an angle.