Controlling nuclear magnetic resonance with a micromechanical oscillator
- A novel technique to individually manipulate nuclear spins in integrated devices -
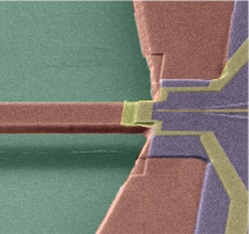
NTT Basic Research Laboratories(NTT) and the National Institute of Advanced Industrial Science and Technology (AIST) have developed a novel device technology to control solid-state nuclear magnetic resonance (NMR)*1 with a micromechanical oscillator. Quantum memory*2 has received much attention in recent quantum technology research for applications to quantum computers, quantum communications, and quantum sensors. Quantum computers are expected to perform ultrafast computation, quantum communications can provide absolutely secure communications protocols, and quantum sensors promise to drastically improve sensor performance. Quantum memory's ability to store quantum superposition states for a long period of time could improve the performance of these quantum technologies. One of the most important candidates for quantum memory is nuclear spin*3 because of its extremely long spin lifetime. NTT and AIST experimentally demonstrated that the NMR frequency in a mechanical oscillator can be controlled by strain induced by its mechanical motion. This method allows us to control the quantum state of a nuclear spin in a desired mechanical element in an integrated array and will play an essential role in the development of solid-state-based quantum memory.
This demonstration was made possible through a collaboration between NTT, which performed device fabrication and measurements, and AIST, which performed data analysis based on precise theoretical modeling. The results were published in “Nature Communications” on August 28, 2018.
This work was partly supported by a MEXT Grant-in-Aid for Scientific Research on Innovative Areas “Science of hybrid quantum systems”.
=> Press Release
=> Nanomechanics Research Group
Background
Nuclear spin is the rotation of an atomic nucleus [Fig. 1(a)], and it shows a precessional motion under a magnetic field [Fig. 1(b)]. Nuclear spin can sustain precessional motion for a long period of time and is expected to be used in various applications, such as quantum memory to store a quantum superposition state and high performance magnetic field sensors. Nuclear magnetic resonance (NMR) is generally used to manipulate the nuclear spin state, where an electromagnetic wave at the NMR frequency is applied under a magnetic field to modify the precessional motion. However, it is hard to use standard NMR techniques for integrated devices because the magnetic field and electromagnetic wave are simultaneously applied to all the devices. Therefore, a novel method to manipulate nuclear spins in individual devices is highly demanded.
Achievement
The NMR frequency is highly sensitive to the strain applied in a solid, and this property was used to locally manipulate nuclear spins in this experiment. Artificially generating strain at a desired position in a material allows the control of NMR in individual integrated devices. A micromechanical oscillator*4 fabricated using state-of-the-art nanofabrication technology was used to locally generate large strain, and the NMR frequency was controlled by oscillating strain by the resonator's mechanical motion. As a result, we observed not only the modulation of the NMR frequency but also a novel kind of NMR called sideband*5 resonance, which were induced by the mixed effect of electromagnetic waves and oscillating strain.
Experimental details
The mechanical oscillator [Fig. 2(a)] has the shape of a doubly clamped beam*6 and was fabricated by processing GaAs, which is a piezoelectric*7 semiconductor. The mechanical oscillation can be electrically induced through the piezoelectric effect. The motion generates periodic strain at the clamping point [Fig. 2(b)] and modifies the dynamics of nuclear spins in the strained region. In the experiments, we observed not only a shift in the NMR frequency (Fig. 3) but also sideband resonances, where the resonance was confirmed also at two shifted frequencies: blue- and red-detuned from the original NMR frequency (Fig. 4). The theoretical calculation taking into account the influence of oscillating strain on nuclear spin dynamics showed good agreement with the experimental results, proving that the observed phenomenon can be well explained by the effect of mechanical oscillation.
Technical features
- Strain in a crystal modifies the atomic lattice structure surrounding the nuclear spins to alter the nuclear spin state. The interaction between nuclear spins and an oscillating strain wave in solid, i.e., an acoustic wave, has been known as acoustic NMR for more than 50 years. However, controlling the NMR frequency requires an acoustic wave with extremely large amplitude, which is difficult to generate in conventional device architecture. In this study, our novel method of using a micromechanical oscillator made it possible to generate such high amplitude of oscillating strain. Owing to this breakthrough, the frequency modulation and sideband NMR resonances were successfully demonstrated for the first time.
- The sideband NMR resonance is a new phenomenon that can be explained by the combined effects of nuclear spin resonance and oscillating strain. The use of novel kinds of resonances allows the manipulation as well as the state readout of nuclear spins in a desired quantum device. The device platform can be applied for multiplexing and integrating quantum sensors and quantum memories, where quantum information is stored as nuclear spins states.
Outlook
The mechanical oscillators are fabricated using semiconductor nanofabrication technology and can be integration on a tiny semiconductor chip. We can utilize this scheme as a novel platform to integrate quantum memories and sensors by demonstrating nuclear spin control in individual arrayed devices.
Publication information
"Dynamical coupling between a nuclear spin ensemble and electromechanical phonons"
Yuma Okazaki, Imran Mahboob, Koji Onomitsu, Satoshi Sasaki, Shuji Nakamura, Nobu-Hisa Kaneko & Hiroshi Yamaguchi
Nature Communicationsvolume 9, Article number: 2993 (2018)
Glossary
*1 ... Nuclear magnetic resonance (NMR)
The axis of nuclear spin shows precessional motion when a magnetic field is applied along the tilt direction. This precession is similarly observed in the motion of a rotating top under a gravitational force. The precessional motion of nuclear spin has a proper frequency specified by the kind of atomic nucleus, and the property can be used to identify the material by measuring the resonance. The method to characterize the resonance frequency of nuclear spin precession by applying a radio-frequency electromagnetic wave is called nuclear magnetic resonance (NMR). NMR is a versatile method for identifying materials in various applications. In particular, magnetic resonance imaging (MRI) is a powerful way to obtain cross-sectional images of the human body and widely used in medical diagnostics.
*2 ... Quantum memory
Quantum memory is a device which can temporally store a quantum mechanical superposition state. It has recently been the focus of much attention in the development of quantum computers and quantum key distribution. In quantum memory, one of the most important requirements is a long life time of stored quantum states, and nuclear spin is one of the most promising physical systems in a solid-state architecture. Nuclear spin has an extremely small magnetic moment, which is typically 1000 times smaller than that of electron spin. Consequently, the influence of external electromagnetic noise sources on the nuclear spin is small and quantum states have a very long life time.
*3 ... Nuclear spin
Materials are composed of atoms as their building blocks, and an atom is composed of an atomic nucleus at its center and electrons orbiting it. An atomic nucleus is about 1000 times smaller than an atom, i.e. the diameter of electron orbits, but the nucleus has its own rotational motion like the earth and moon. The rotational motion is called nuclear spin. The rotational speed is fixed, specified by the species of nuclear spin, but the rotational axis is altered by the interaction with the environment, such as with external magnetic and electric fields, as well as the magnetic moments generated by electrons.
*4 ... Mechanical oscillator
A mechanical oscillator is an artificially fabricated structure that has its own mechanical vibration mediated by periodic elastic deformation. Some musical instruments such as the bell and vibraphone are macroscopic mechanical oscillators. It has recently becomes possible to fabricate ultra-small oscillators integrated on a semiconductor chip using state-of-the-art nanofabrication technologies. The integrated devices are called micro-electromechanical systems (MEMS), and various applications such as actuators and sensors have been developed. One of the typical shapes of a mechanical oscillator is a doubly clamped beam, which is similar to a bridge or a plate of vibraphone.
*5 ... Sideband
A Sideband is the frequency generated by mixing two periodic signals observed at their sum or difference frequencies. One example can be found in amplification circuits for radio frequency signals, where the high-frequency signal is converted to a lower frequency by mixing it with a signal generated by a local oscillator. The generation of a sideband is therefore useful for converting the signal frequency and can also be used to convert quantum states between different physical media in recent quantum information technologies. In this study, the NMR frequency was converted into different sideband frequency by mixing the effects of an electromagnetic wave and periodic strain.
*6 ... Doubly clamped beam
One of the most widely used micro-mechanical oscillators fabricated by using state-of-the-art nanofabrication technology. It consists of a thin bar, both sides of which are fixed (Fig. 2(b)), and vertical motion is induced. The motion generates large strain at the clamping areas, where NMR frequency can be controlled.
*7 ... Piezoelectric effects
When a voltage is applied to an ionic material, the material is expanded or compressed due to the electrostatic deformation of its atomic structure. This effect is called piezoelectricity. It is also possible to reversely generate a voltage by applying a strain on the material. The effect can be used to actuate mechanical oscillation and to electrically detect the elastic deformation of a mechanical element.