Superconducting circuit containing Josephson junctions is one of the
promising candidates as a quantum bit (qubit) which is an essential ingredient
for quantum computation [1]. A three-junction flux qubit [2] is one of
such candidates. On the basis of fundamental qubit operations [3,4], the
cavity QED like experiments are possible on a superconductor chip by replacing
an atom with a flux qubit, and a high-Q cavity with a superconducting LC-circuit
(Fig.1). By measuring qubit state just after the resonant interaction with
the LC harmonic oscillator, we have succeeded in time domain experiment
of vacuum Rabi oscillations, exchange of a single energy quantum (photon),
in a superconducting flux qubit LC harmonic oscillator coupled system [5].
The observed vacuum Rabi frequency 140 MHz is roughly 3×103 (1×107) times larger than that of Rydberg (ordinary) atom coupled to a single
photon in a high-Q cavity [6]. This is a direct evidence that a strong
coupling condition can be rather easily established in the case of macroscopic
superconducting quantum circuit. It is explained by the reasons that the
circuit is huge compared with the atomic scale and also the super-current
of µA order flows in the qubit. We have also obtained evidence of level
quantization of the superconducting LC circuit by observing the change
in the quantum oscillation frequency when the LC circuit was not initially
in the vacuum state (Fig.2). We are also considering this quantum LC oscillator
as a quantum information bus by sharing it with many flux qubits, then
spatially separated qubits can be controlled by a set of microwave pulses
just like the method used in the quantum optics.
[1] F. Wilhelm and K. Semba, in "Physical Realizations of Quantum Computing: Are the Divincenzo Criteria Fulfilled in 2004?", (World Scientific Publishing Company; April, 2006)
[2] J. E. Mooij et al., Science 285 (1999) 1036.
[3] T. Kutsuzawa et al., Appl. Phys. Lett. 87 (2005) 073501.
[4] S. Saito et al., Phys. Rev. Lett. 96 (2006) 107001.
[5] J. Johansson et al., Phys. Rev. Lett. 93 (2006) 127006.
[6] J. M. Raimond, M. Brune, and S. Haroche, Rev. Mod. Phys. 73(2001) 565.
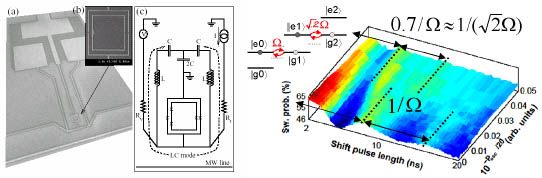 |
Fig. 1. |
(a) Scanning Electron Micrograph of the sample (qubit, SQUID, and an on-chip
LC harmonic oscillator). (b) close-up view of a qubit and a SQUID detector
(c) Equivalent circuit of the sample. |
|
|
Fig. 2. |
Rabi oscillations as a function of the duration of the flux bias shift
pulse and the amplitude of an LC-oscillator weak resonant pulse. The lowest
two quantized Rabi periods are observed. |
|
|