A new method for the artificial assembly of micro-scale biological tissues
- Enabling the formation of arbitrary 3D structures that are highly biocompatible and flexible, and the encapsulation of cells for long-term cultures -
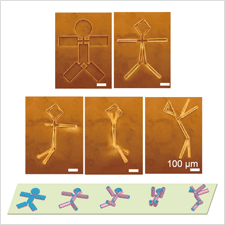
NTT Basic Research Laboratories has developed a method for the reconstruction of tiny biological tissues like cardiac muscle and nerves at scales ranging from microns to centimeters. This is done by incorporating cells into 3D structures fabricated entirely out of highly biocompatible polymer films, and culturing these cells for a prolonged period of time.
In this research, we successfully fabricated 3D structures by forming layers of silk fibroin gel*1 and parylene*2 (two types of polymer film that are widely used as implantation materials) with varying shapes and thicknesses that could be assembled into arbitrary 3D shapes. If 3D structures can be freely fabricated from materials that are biocompatible and highly flexible, then we can expect this technique to find applications such as flexible substrates for cell cultures and implantable elements that fit to the surface shape of biological tissues.
We have also discovered that it is possible to form micro-scale artificial self-organizing structures by implanting cells inside 3D structures where they can be cultivated for long periods of time so that the cells gradually aggregate within these structures. For example, we have succeeded in the reconstruction of myocardial fibers by culturing myocardial cells, and in the production of microscopic neural tissue by culturing hippocampal cells. Our findings are expected to lead to fundamental regenerative medicine techniques using stem cells, and the creation of new biological interfaces such as functional bio-implant devices that can respond to external electrical or magnetic fields.
This work was published online in the UK scientific journal “Scientific Reports” at 10 am (UK time) on 22nd December 2017.
=> Press Release
=> Molecular and Bio Science Research Group
Background of the research
Techniques for the artificial creation of structures that are close to a single type of biological tissue by assembling cells in a three-dimensional structure are indispensable in a wide range of fields including drug discovery, regenerative medicine, and transplantation therapy. Methods that have hitherto been widely used for the formation of cell clusters (aggregates) include the manipulation of individual cells to incorporate them into templates with minute channel structures, and the use of inkjet printers to deliver cells in a predetermined arrangement. However, in these methods, it is difficult to increase the accuracy of the three-dimensional shape, and it is not possible to fabricate cell aggregates in arbitrary shapes. On the other hand, in a method that uses thin films of inorganic materials like silicon or gallium arsenide, the difference in elastic coefficients of the materials is used to induce a strain in the thickness direction, thereby enabling the self-organized assembly of any 3D structure (self-assembly). In this method, it is known that shape control can be performed with a precision of a few hundred nanometers. However, since the materials used in the thin film fabrication process lack biocompatibility, it is very limited in terms of applications involving biological materials such as cells and biological tissues. If soft materials such as highly biocompatible hydrogels*3 can be used to fabricate arbitrary 3D structures, then it should be possible to fabricate interfaces that are kind to living tissues such as cell culture substrates and implantable devices that fit to the surface shape of biological tissue.
Research results
We focused on the principles of self-assembly, and by applying them to biocompatible polymer materials, we discovered a method for bringing about the spontaneous assembly of 3D shapes configured entirely from polymer materials. Furthermore, by implanting and cultivating cells inside these 3D structures during the self-assembly (Fig. 1), we succeeded in the creation of artificial structures resembling living tissue using 3D structures as templates.
Description of the experiment
We used two types of polymer thin film materials in this study — a silk fibroin gel derived from the purification of silk fibers, and poly (p-xylene) (parylene). In addition to these polymers, we produced on a substrate a polymer film comprising three types of film to which a film of calcium alginate gel*4 had been added as a sacrificial layer. Using photolithographic techniques, the thin films laminated with polymers can be micro-patterned into arbitrary 2D shapes (Fig. 2).
The calcium alginate gel can be dissolved using a chemical such as ethylenediaminetetraacetic acid (EDTA).*5 This leaves behind a thin film of silk fibroin gel and parylene that can be freed from the substrate by a non-cytotoxic reaction. After adding EDTA, we observed that the calcium alginate gel is dissolved away working gradually inwards from the periphery of the thin film, and at the same time, the thin film deformed into a 3D structure.
Technical point: 3D self-assembly method that is compatible with cells
- The 3D structures can be controlled by designing the thin-film pattern
In this study, the phenomenon whereby thin films self-assemble into 3D structures originates from differences in the elastic coefficients of two thin film layers. These form internal stress gradients in the film thickness direction, resulting in a spontaneous deformation mechanism that can be used to build these structures. For example, a rectangular thin film bends in the direction of its short side, allowing it to be assembled spontaneously into a tubular structure (Fig. 3 (a)). We have found that this radius of curvature is governed by the elastic modulus of the polymer, the thickness of the thin film, and the two-dimensional shape of the thin film. We have also verified that it is possible to form arbitrary 3D structures from 2D shapes, such as spherical structures from radial patterns, and stick-figure structures from rectangular patterns joined by hinges (Fig. 3(b), Video 1). - Cells can be encapsulated without exposure to toxicity
The method we used to eliminate the calcium alginate layer does not expose the cells to toxicity, so this self-assembly process is able to encapsulate multiple cells into 3D structures at the same time (Fig. 4, Video 2). The encapsulated cells can be used not only with cell culture lines that are generally used in biological research, but also with primary culture cells that have been directly isolated from rat tissue. It is also possible to create cell clusters of any shape by controlling the density of the encapsulated cells and the shape of the three-dimensional template structure.
When cells encapsulated in a tubular structure have been cultured for about a week, they change into living tissue-like structures with unique cell functions. In this study, using first-stage cardiomyocytes as an example, we demonstrated that cells can aggregate inside a tubular structure and then form the structure of a single microscopic myocardium fiber (Fig. 5, Video 3). We also saw the cardiomyocytes in this myocardium fiber beating synchronously, and we measured the intercellular interactions that give rise to intracellular calcium fluctuations with the same period as the pulse. In addition, by culturing first-stage hippocampal cells inside a tubular structure, we were able to form cell clusters by combining cells together during long-term culturing, after which we observed neurites growing inside and outside the structure (Video 4). In a cell cluster made from these hippocampal cells, cells connected by neurites respond simultaneously to external stimuli, demonstrating the intercellular interaction in reconstructed microscopic nerve tissue. Since this tubular structure is made with materials and processes that do not exhibit toxicity to cells, it is possible to culture cells inside this structure for long periods of time. - Micro-roll handling technology
It is also possible to use glass capillary tubes to capture cell masses formed inside the structures, and move them to other locations as required (Video 5). It is therefore possible to form artificial neural circuits by bringing together tubes containing encapsulated cells and allowing the cells to interconnect, and it is also possible to form any three-dimensional structure such as arbitrary biological tissues. We expect that this technique can be applied to the encapsulation of various kinds of cells to produce biointerfaces that perform various functions, such as embedded devices in biological tissues.
Future prospects
This method enables groups of encapsulated cells to be freely manipulated by forming any 3D shape spontaneously from highly biocompatible polymer materials. It is therefore capable of forming scaffolding structures for use in fields such as regenerative medicine and the behavior analysis of single cells. Furthermore, by adding functions such as conductivity and magnetic sensitivity to the polymer materials used in this research, it could be possible to develop new forms of biological interface for embedded devices with enhanced functions.
Publication details
Authors: Tetsuhiko F. Teshima, Hiroshi Nakashima, Yuko Ueno, Satoshi Sasaki, Calum S. Henderson, and Shingo Tsukada
Title: Cell Assembly in Self-foldable Multi-layered Soft Micro-rolls
Journal title: Scientific Reports
Publication date: 22nd December 2017, 10 am (UK time)
Glossary
*1 ... Silk fibroin gel
Silk fibroin is an essential protein that makes up about 70% of the protein in silk. It has a fibrous structure, and is thought to contribute to the high tensile strength, stretchability and water absorption qualities of the material. It is also highly biocompatible, and is used in applications such as cell culture substrates and artificial skin that promotes tissue regeneration. Silk fibroin gel consists of silk fibroin that has been dissolved in an aqueous solution, diluted and then formed into a gel.
*2 ... Polyparaxylene (Parylene)
A polymer with a linear crystalline structure that is a good insulator and acts as a strong barrier against moisture and chemical substances. In addition, it has excellent heat resistance and ultraviolet resistance, is highly biocompatible, and is used as a coating agent in a wide range of industrial fields including medical care.
*3 ... Hydrogel
A collective name for polymer substances that consist of an intertwined network of macromolecules with water held in the gaps between these molecules. This three-dimensional microscopic network structure becomes swollen when water molecules are introduced, resulting in a material with high fluidity and viscoelasticity.
*4 ... Calcium alginate gel
Alginic acid is a polysaccharide that can be extracted from seaweeds such as kelp and sea mustard. It consists of two types of monosaccharide (mannuronic acid and guluronic acid) joined in a straight chain. A calcium alginate gel is formed when these saccharides are cross-linked by calcium ions and swell up with the addition of water. When this material is formed between a silk fibroin gel and parylene laminated thin film and a glass substrate, it can be removed by ethylenediaminetetraacetic acid. This allows the thin film to be liberated from the glass substrate without harming any cells.
*5 ... Ethylenediaminetetraacetic acid (EDTA)
Ethylenediaminetetraacetic acid is a chelating agent commonly known as EDTA. It binds strongly with divalent ions such as calcium to form a complex. It is widely used in industry for the removal of heavy metals from fiber pulp, medicines and foods, and is used in the biological field for the removal of calcium and the deactivation of enzymes. In this study, it is used to remove calcium ions from the calcium alginate layer (*4), thereby dissolving the layer and releasing the thin film.
Video Links
Video 1: Construction of three-dimensional polymer thin films
Video 2: Damage-free cell encapsulation
Video 3: Reconstruction of microscopic muscle tissue using cardiomyocytes
Video 4: Reconstruction of nerve fibers using hippocampal cells