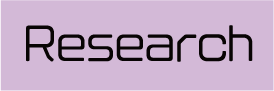
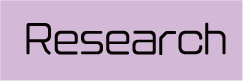
Research
Our group pursues innovative cutting-edge theoretical research in quantum physics and quantum information processing. We are interested in how it can be applied to the real-world development of quantum-enabled technologies. The main areas of our research are:
Foundations of Quantum Mechanics
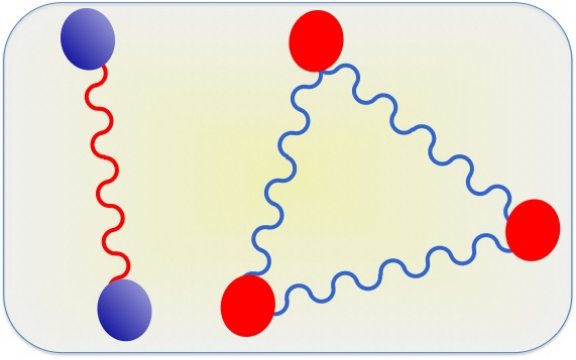
The foundation of quantum theory has been a great source of stimulation to the development of quantum information science. Conversely, the insight obtained in quantum information science can help deepen our understanding of the foundational aspects of quantum theory itself.
We try to illuminate the weird nature of quantum theory, especially in understanding quantum randomness and nonlocal features induced by entanglement. Our recent work includes:
We try to illuminate the weird nature of quantum theory, especially in understanding quantum randomness and nonlocal features induced by entanglement. Our recent work includes:
- Yusuke Hama, William J. Munro, and Kae Nemoto, Relaxation to Negative Temperatures in Double Domain Systems, Phys. Rev. Lett. 120, 060403 (2018).
- G. C. Knee, K. Kakuyanagi, M. Yeh, Y. Matsuzaki, H. Toida, H. Yamaguchi, S. Saito, A. J. Leggett and W. J. Munro, A strict experimental test of macroscopic realism in a superconducting flux qubit, Nature Communications 7, 13253 (2016).
- Masato Koashi, Koji Azuma, Shinya Nakamura, and Nobuyuki Imoto, Does "quantum nonlocality without entanglement" have quantum origin?, arXiv:1303.1269 (2013).
The Quantum Internet
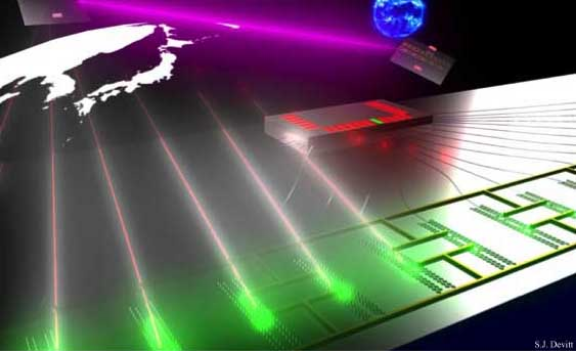
TThe quantum internet is a quantum version of the current Internet, which is considered to be an ultimate form of networks allowed by the principles of quantum mechanics.
Analogously to the conventional networks, the quantum internet is composed of information processing nodes and communication channels. However, these nodes and channels are based on purely quantum technologies such as a quantum computer and a quantum channel. As a result, the quantum internet has already held promise for accomplishing quantum teleportation and unconditionally secure communication freely between arbitrary clients all over the globe, as well as the simulation of quantum many-body systems.
Our group works out to understand the full potential of the quantum internet. In particular, we challenge to answer the following fundamental questions: What kind of novel application, intractable by the conventional internet, would be possible? What is the ultimate performance of quantum internet protocols? Several examples of our recent work include:
Analogously to the conventional networks, the quantum internet is composed of information processing nodes and communication channels. However, these nodes and channels are based on purely quantum technologies such as a quantum computer and a quantum channel. As a result, the quantum internet has already held promise for accomplishing quantum teleportation and unconditionally secure communication freely between arbitrary clients all over the globe, as well as the simulation of quantum many-body systems.
Our group works out to understand the full potential of the quantum internet. In particular, we challenge to answer the following fundamental questions: What kind of novel application, intractable by the conventional internet, would be possible? What is the ultimate performance of quantum internet protocols? Several examples of our recent work include:
- Luca Rigovacca, Go Kato, Stefan Baeuml, M. S. Kim, W. J. Munro, and Koji Azuma, Versatile relative entropy bounds for quantum networks, New J. Phys. 20, 013033 (2018).
- Koji Azuma, Akihiro Mizutani, and Hoi-Kwong Lo, Fundamental rate-loss trade-off for the quantum internet, Nature Commun. 7, 13523 (2016).
- Koji Azuma, Kiyoshi Tamaki and William J. Munro, All-photonic intercity quantum key distribution, Nature Commun. 6,10171 (2015).
- Koji Azuma, Kiyoshi Tamaki, and Hoi-Kwong Lo, All photonic quantum repeaters, Nature Commun. 6, 6787 (2015).
- W. J. Munro, A. M. Stephens, S. J. Devitt, K. A. Harrison and Kae Nemoto, Quantum communication without the necessity of quantum memories, Nature Photonics 6, 777 - 781 (2012).
Quantum Computation and Simulation
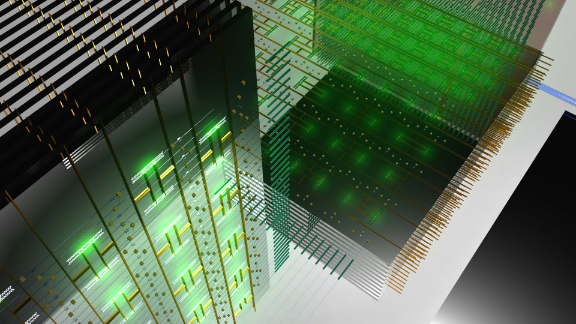
The development of technologies based on the principles of quantum mechanics are likely to be one of the core foundations upon which the twenty-first century will rely. In fact, these resources allow tasks such as unconditionally secure communication and the prime factorization of large integers, which are of utmost importance for technological applications.
In addition, as Feynman proposed in 1982, we might be able to use quantum systems that are highly controllable, to simulate other quantum systems. In the near future, we will have access to Noisy Intermediate-Scale Quantum technology (NISQ), which will open a new avenue in the field of quantum simulation of manybody systems. Even for a modest number of interacting qubits, this task is is extremely difficult, if not impossible to achieve with a classical computer. Arrays of superconducting qubits constitutes one of the most important platforms for implementation of quantum simulators. This technology allows one to exploit the interactions between microwave photons to simulate highly-correlated and topological states of matter, even in the presence of disorder. The possible applications are broad, ranging from quantum metrology, imaging and sensing, to quantum chemistry and quantum computation.
We are investigating how to create quantum information processing devices such as simulators and computers. Several examples of our recent work include:
In addition, as Feynman proposed in 1982, we might be able to use quantum systems that are highly controllable, to simulate other quantum systems. In the near future, we will have access to Noisy Intermediate-Scale Quantum technology (NISQ), which will open a new avenue in the field of quantum simulation of manybody systems. Even for a modest number of interacting qubits, this task is is extremely difficult, if not impossible to achieve with a classical computer. Arrays of superconducting qubits constitutes one of the most important platforms for implementation of quantum simulators. This technology allows one to exploit the interactions between microwave photons to simulate highly-correlated and topological states of matter, even in the presence of disorder. The possible applications are broad, ranging from quantum metrology, imaging and sensing, to quantum chemistry and quantum computation.
We are investigating how to create quantum information processing devices such as simulators and computers. Several examples of our recent work include:
- Kae Nemoto, M. Trupke, S. J. Devitt, A. M. Stephens, B. Scharfenberger, K. Buczak, T. Nobauer, M. S. Everitt, J., Schmiedmayer, and W. J. Munro, Photonic Architecture for Scalable Quantum Information Processing in Diamond, Phys. Rev. X 4, 031022 (2014).
- S. J. Devitt, A. M. Stephens, W.J Munro and Kae Nemoto, Requirements for fault-tolerant factoring on an atom-optics quantum computer, Nature Communications 4, 2524 (2013).
- Koji Azuma, Hitoshi Takeda, Masato Koashi, and Nobuyuki Imoto, Quantum repeaters and computation by a single module: Remote nondestructive parity measurement, Phys. Rev. A 85, 062309 (2012).
Hybrid Quantum Systems
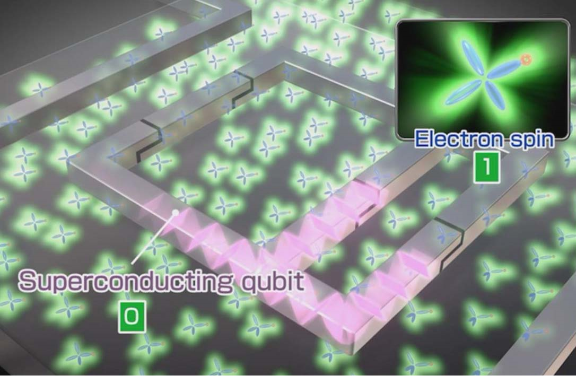
It is now considered that no single type of physical system possesses all the required attributes for quantum information processing tasks such as computation, communication and sensing.
Systems that benefit from an excellent ability to perform multi-qubit gates tend to have short coherence times, while those that have long coherence times are limited in their ability to perform multi-qubit gates. Hybridization of quantum systems from each class, however, allows one to exploit the best properties of the individual systems. Nitrogen-vacancy centers in optical cavities possess an extremely long lived nuclear spin, as well as an electron spin which couples to optical fields, allowing natural remote two-qubit gates to be achieved. Such a hybrid approach naturally enables distributed information processing, where remote nodes are networked together. Superconducting circuits and electron spin ensembles are another example. Here the superconducting qubits provide easy manipulation and processing of information, while a spin ensemble (having long storage times) can act as a quantum memory.
Our group theoretically explores various aspects of these hybrid systems including how they can be realised and used as well as modelling them. Several recent papers include:
Systems that benefit from an excellent ability to perform multi-qubit gates tend to have short coherence times, while those that have long coherence times are limited in their ability to perform multi-qubit gates. Hybridization of quantum systems from each class, however, allows one to exploit the best properties of the individual systems. Nitrogen-vacancy centers in optical cavities possess an extremely long lived nuclear spin, as well as an electron spin which couples to optical fields, allowing natural remote two-qubit gates to be achieved. Such a hybrid approach naturally enables distributed information processing, where remote nodes are networked together. Superconducting circuits and electron spin ensembles are another example. Here the superconducting qubits provide easy manipulation and processing of information, while a spin ensemble (having long storage times) can act as a quantum memory.
Our group theoretically explores various aspects of these hybrid systems including how they can be realised and used as well as modelling them. Several recent papers include:
- Andreas Angerer,Stefan Putz, Dmitry O. Krimer, Thomas Astner, Matthias Zens, Ralph Glattauer, Kirill Streltsov, William J. Munro, Kae Nemoto, Stefan Rotter, Jorg Schmiedmayer and Johannes Majer, Ultralong relaxation times in bistable hybrid quantum systems, Sci. Adv. 3, e1701626 (2017)
- Stefan Putz, Andreas Angerer, Dmitry O. Krimer, Ralph Glattauer, William J. Munro, Stefan Rotter, Jorg Schmiedmayer and Johannes Majer, Spectral hole burning and its application in microwave photonics, Nature Photonics 11, 3639 (2016)
- X. Zhu, Y. Matsuzaki, R. Amsuss, K Kakuyanagi, T. Shimo-Oka, N. Mizuochi, Kae Nemoto, K. Semba, W. J. Munro and S. Saito, Observation of dark states in a superconductor diamond quantum hybrid system, Nature Commun 5, 3424 (2014).